
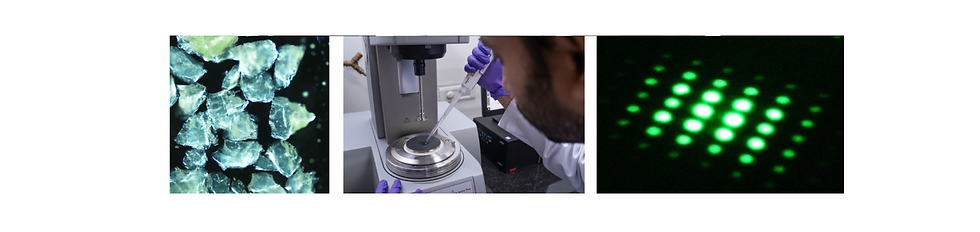
Overview
Current research activities at our laboratory revolve around understanding the collective dynamics of self powered micro/nanomotors and also realizing their application potential towards sensing, delivery and useful energy harvesting. We study and characterize the behaviors of active materials under different experimental conditions and simultaneously develop theoretical models and simulations to gain deeper insights into the observed phenomena.
Specifically, we seek to address the following questions: Which are the most efficient mechanisms through which micro/nanomotors can uptake energy from the environment? How can motion control be established for these motors, both at the single particle and collective level, to achieve specific applications pertaining to transport and delivery? How does interparticle communication in an active assembly influence their emergent dynamics and to what degree can these behaviors be tuned on demand? What are the ranges and magnitudes of reaction induced force fields? How optimal navigation of swimmers can be achieved under noisy environments? Are there generic principles based on which active swimmers interact with their surroundings, independent of their sizes and propulsion mechanisms? And most importantly, how can these active motors be interfaced with biological systems without causing toxicities and other adverse effects?
Focus Areas
Enzyme-Based Active Matter in Crowded Environments
Using complementary theory and experiments, we investigate the behavior of biological catalysts, such as enzymes, in crowded and inhomogeneous conditions to gain insights into the fundamental processes governing biochemical reactions in intracellular environments.
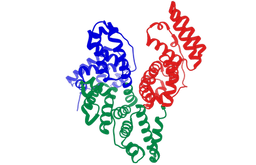
Why Study Enzymes in Crowded Environments?
Enzymes are the workhorses of biological systems, orchestrating an incredible array of chemical transformations necessary for life, inside cellular environments which are crowded with macromolecules, ions, and other cellular components. This crowdedness can significantly impact their kinetics and dynamics, altering their ability to transduce mechanical fluctuations and interact with their surroundings. Active fluctuations generated by enzymes during reactions also play key roles in governing colloidal dynamics and interactions at low Reynolds number.
Understanding how enzymes are able to generate forces and function within crowded environments is not only intriguing from a fundamental standpoint but also holds immense practical significance. Engineered enzyme systems find applications in various fields, including biotechnology, medicine, and environmental science. By elucidating the behaviors of enzymes in complex intracellular conditions, we aim to enhance the efficiency and applicability of enzyme-based technologies and deepen our understanding of fundamental biological processes.
Microbubbles as Active Matter
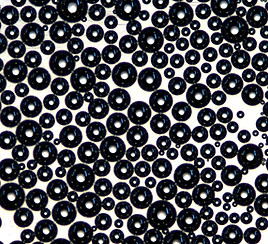
Bioactive microbubbles are enzyme driven miniature bubbles that are encapsulated with molecular cargo such as drugs, genes, and imaging agents. Microbubbles offer immense opportunities across a wide range of applications such as enhanced contrast imaging and targeted drug delivery, making them a subject of intense scientific interest. Imparting chemical activity to these bubbles is expected to open up a whole new research area in molecular biophysics pertaining to targeted transport and delivery of materials under crowded conditions. Due to their unique 'vanishing' capabilities after achieving desired goals in vivo, these microbubble motors hold greater promise in designing biocompatible drug delivery carriers compared to synthetic polymer motors. Our research delves into the behavior and collective dynamics of these tiny, protein shelled microbubbles for possible applications in delivery and theranostics.
​
​
Why Study Bioactive Microbubbles?
Microbubbles are widely used as contrast agents in medical imaging techniques as well as carriers for therapeutic agents, for targeted drug delivery to specific tissues or cells. By studying the dynamics of bioactive microbubbles, we aim to enhance their motility for enhanced transport of molecular cargo within dense and complex cellular environments. By investigating and optimizing their behavior under in vivo conditions, we seek to establish control over drug loading, release kinetics, and targeting strategies, ultimately improving the efficacy and safety of drug delivery strategies. Beyond these applications, bioactive microbubbles also hold potential for therapeutic interventions such as sonoporation (using ultrasound to temporarily increase cell membrane permeability) and targeted therapy. Understanding their dynamics under various external conditions is therefore essential for maximizing therapeutic outcomes while minimizing adverse side effects.
​
Influence of Active Matter Dynamics over Intracellular Transport and Delivery
Intracellular transport is a complex phenomenon essential for various cellular processs, including signal transduction, organelle positioning, and molecular trafficking. We aim to harness non-thermal fluctuations generated by biomolecular active matter in modulating the movement of biomolecules and nanoparticles within living cells, which is expected to have important implications for biomedicine and therapy.

Why Study Intracellular Transport and Delivery?
Cells are dynamic systems teeming with molecular motors, cytoskeletal filaments, catalytic enzymes and other active components that collectively drive intracellular transport processes. By studying the influence of biomolecular activity over materials transport in and around cellular milieu, we gain insights into the fundamental principles governing cellular organization and function. Moreover, understanding how active matter dynamics modulate nanoparticle transport within cells can enable us to design more effective drug delivery strategies and enhance their therapeutic efficacy.
​
Our Funding Agencies
_JPG.jpg)
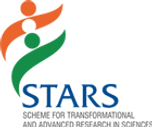
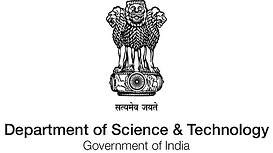
